AMHERST — Greg Grason draws inspiration from the wings of butterflies and birds.
As a researcher and professor of polymer science at the University of Massachusetts Amherst, he and his team look to nature when thinking about how proteins assemble themselves into the cells and tissues of living organisms.
“Nature has figured out how to do it,” said Grason, speaking of this process. “So we are trying to mimic those structures from the bottom up.”
According to Grason, the ability to design synthetic building blocks would open a door to many real-world possibilities.
“People will say that DNA is not just the secret of life, but you can use it as a building block,” said Grason. He likens it to “DNA origami.”
He says the research could lead to applications in a variety of technologies, from energy to human health.
Grason compares this self-assembling to what happens when the body suffers a cut. The body naturally calls up certain types of proteins, called fibrin, that self-assemble to reinforce the cut. The proteins are tough enough to reinforce the wound, but flexible enough to allow movement. Over time, as the wound heals, the proteins either disassemble or degrade.
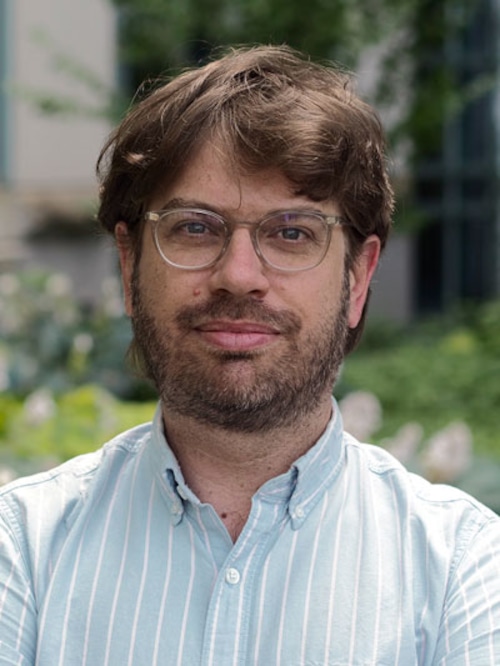
Researcher Greg Grason of the University of Massachusetts Amherst. (UMass photo)Staff
Possible use
In their work at UMass, researchers have been drawing upon the reflective colors on bird feathers, butterfly wings and beetles. Three-dimensional networks of proteins bear a pattern that allows light to reflect off of them, Grason said, causing them to look blue or green.
Grason said this research could result in a coating that could be painted or sprayed on to provide an iridescent color — which could be used for light control on solar cells or fibers for internet connection. Due to the self-assembling nature of the coatings, Grason said it could be a low-cost and low-energy option.
Another possibility that would require more research is to use these self-assembling proteins as building blocks in tissue replacement. This could be a less-invasive option than what is now available, as the proteins could be injected with a micro-needle rather than in a surgical procedure.
Along with colleagues at Brandeis and Syracuse universities, the UMass researchers are trying to understand the engineering principles needed to mimic the types of structures found in nature. They are looking to models from the natural world, even the microscopic one, that could guide the creation of ways to transform the shape of DNA.
“You can take the genetic sequence of DNA and techniques for synthesizing short DNA strands to get it to fold up into different complicated shapes,” said Grason.
That’s where the DNA origami comes in.
He said that once the proteins assemble, there is no reason for it to stop, but regulating this on a larger scale can pose a challenge.
Grason compared proteins to “bricks” that build everyday structures. He said the “mortar” takes the form of “attractive” interactions, such as positive and negative electrical charges arranged on the proteins. In nature, though, the bricks stack themselves, and stop once the structure has reached the right size.
“The goal,” said Grason, “is to do the same thing, but instead of using the ‘protein bricks’ that nature has evolved, form them from some chemical synthesis that is human engineered.”
Art of mimicry
Grason and his team of researchers are trying to understand why some “bricks” are stickier than others. They are also trying to determine how to encode the bricks to know when to stop building, just as in nature.
While Grason has been at UMass since 2007, he has been working on this collaboration with Brandeis since 2018 with his team in the Grason Research Group.
The research started when his collaborators at Brandeis started mimicking the assembly of protein shells that surround viruses.
“One of our collaborators was thinking how you can use these DNA origami shells in a way to trap viruses in them by coating antibodies that can bind herpes virus on the inside of those self-assembled structures,” said Grason.
“Their work showed that you could grow larger shells using the smallest number of different types of ‘bricks,’ or in their case, programmable DNA-based particles.”
Grason’s group then figured out that you could adapt the economy of design, but switch the type of assembly from spherical shells to what he called “interconnected spongy labyrinths.”
This allows the researchers to take advantage of unique optical properties, similar to those found in butterfly wings that would provide real-world reflective coatings. They tested a model that determined the right conditions for these assembly types, based on computer simulations.
The researchers note that the shape of the synthetic triangles is important.
Grason explained that if they had what’s known as a “positive” curvature, they would close in on themselves, like a ball. A negative curvature is key to provide a shape where one side curves up and the other curves downwards, resulting in a “tubular” shape that can be repeated in a pattern.
According to Grason, it’s important to control not only which particle likes to sit next to another, but also control how flexible those interactions are. They can’t be too stiff or too flexible. Grason compares this work to building LEGOs with DNA.
“Even though we’re talking about small molecules,” said Grason, “a lot of the rules are geometrical.”